By: Conor O’Sullivan | December 2014
Abstract
Microplastics (> 1μm to < 1 mm) are a pollutant waste product of the industrial, and consumer-driven economies of the 20th and 21st centuries. The prevalence of microplastics in the world’s oceans and on the world’s continents is directly related to the unchecked production, consumption and improper disposal, decomposition and subsequent leaching of plastic particles from macroplastic (> 1 mm) items, such as plastic pellets or plastic bags. This is cause for great ecological and human health concern at all geographical scales. Research literature was gathered from electronic research databases to develop an understanding of how such pollution is effecting marine ecosystems, and the feasibility of adapting to and mitigating both current and future damage at an international level. This paper will serve as a literature review of research results across relevant disciplines of marine ecology, biology, law and policy, and technology in hopes of accurately assessing the effects of microplastics as an international pollution issue and how we may attain and sustain the health of our world’s oceans for the future. Results remain inconclusive as to how feasible detoxifying the world’s oceans may be, as concerted efforts on all scales of governments worldwide to mitigate the issue have not been and are still not commonplace, though smaller projects show some promise. Seemingly, the filtering of microscopic plastic particles is costly, inefficient and the damage may be here to stay for quite some time, even with concerted effort at all levels of geographic scale.
Keywords: Microplastics, production, decomposition, health, mitigation, adaptation
Introduction
Microplastics are the byproduct of physical weathering (i.e. shredding, tearing) and chemical dissolving of larger, or macroplastic (> 1mm) items, such as plastic bags, plastic pens, wrappers, etc. (Andrady, 2011). Microplastics are not universally defined in size but can be defined as being > 1μm to < 1mm in size, and are an ever-growing issue for environmental and human health worldwide as the production and ubiquity of plastic items continues to grow for the sake of human industry and convenience. In recent years microplastics have become an international issue of increasing importance, especially amongst industrialized, coastal nations sharing oceans in which macroplastic items have been found to aggregate, such as in the North Pacific Gyre (Cózar et al., 2014; Larsen, 2014), circulating between the Western coast of America and the Eastern coasts of China, Korea, Japan and Russia. It is no secret that nations which have industrialized, mimicking western models of modernization, use plastics in far greater quantities than unindustrialized or industrializing nations, but determining point-sources of microplastic pollution by specific nations is difficult due to the size of the oceans and under-reporting of plastic debris (Barnes et al., 2009). Previous studies point out the difficulties by which the advent and dispersement of plastics and microplastics pose to clean-up efforts and the development and establishment of effective governmental policy (Barnes et al., 2009; Shaxson, 2009), as well as how resulting microplastics disruptively interact with both the terrestrial and marine habitat environments (Ward & Kach, 2009; Talsness et al., 2009).
The improper discarding of waste materials from human centers of civilization is nothing new, and has been a byproduct of building and maintaining societies for thousands of years. However, in our modern age, the necessities of modern life demand that nearly all products contain plastics and other harmful materials for our convenience, which end up as discarded waste at vastly greater quantities and at a vastly greater rate (Barnes et al., 2009; Thompson et al., 2009). Thus, yet another global issue with a fast-approaching tipping point of exhausting the resilience of ecosystems is actively and carelessly being forced to become the responsibility of current and near-future generations, with documented high risk to marine animal and human endocrinological health (Talsness et al., 2009; Oehlmann et al, 2009). Thus, the ability for removal of both macro and microplastic items from consumption and the world’s oceans is of paramount importance, and is a multifaceted endeavor requiring a sense of urgency and cooperation at county, state and national levels. Fortunately, some important legal actions have been brought into effect at multiple political scales, such as the international MARPOL 73/78 agreements, to limit the amounts of plastics dumped at sea (MARPOL, 1973). Other legislation at smaller scales to limit plastic bag consumption have been enacted, but by only a few cities around the United States of America, as well as the state of California, and the nation of Ireland (Larsen, 2014; Thompson et al. 2009a).
Again however, due to the growing demands of the 21st century economy, the use of plastics is expected to grow in relation to projected global population despite such bans on items such as plastic bags (Andrady, 2011). Regions of the world that rely more heavily on the use of plastic items, which quickly become discarded and make their way into natural habitats, will face issues of ecosystem loss and human health in greater numbers than regions which produce and use less (Talsness et al., 2009). Efficient and progressive technological improvements have allowed for incorporation into large scale clean up projects, with promising results (The Ocean Clean Up, 2014). However, with little effort being put forth into public awareness and the developing and investing into materials which may be able to replace plastics along the same scale of use without major industrial overhaul and a further taxing on the environment (Song et al., 2009), questions and concern remain as to how and if we can mitigate our impacts on the environment. Political unwillingness and lack of urgency towards halting the production of plastics, as well the continued under-funding of clean-up projects and effective filtering technologies only exacerbate the issue, especially if favor in continuing plastics production persists. Technological development may have a long way to go before mitigation is noticeable, suggesting that the effects of microplastics may be longer lasting then currently recognized by heads of state and the general population.
Literature Review
Plastic is and Plastic was – Growth in Production and Use
Over the last century our consumption, and disposal of commercial products has undoubtably increased, with the composition of such products increasing post-use longevity and leading to adverse effects on marine environmental health. Analyses of current literature by Thompson et al., (2009a) highlighted historical and current trends in growth of consumption and disposal of plastic, as well as the health concerns arising from the ubiquity of chemically laden plastic items on land and in the sea. According to Thompson et al., (2009a), since the initial production of consumer plastic items in the 1940’s, production has increased from roughly one million tons a year to upwards of 260 million tons, with similar research estimating production levels ranging between 265 million and 299 million tons per year (Cózar et al., 2014; PlasticsEurope, 2014). They concluded that for daily operations, humanity will continue to become evermore dependent on the uses of plastics in the future, along with the growing population. The research which Thompson and colleagues included in their review also addressed the strategies and current technologies being used to examine the fate of plastics after they have been used, and were quick to point out gaps in policy and oversight as a large factor for why so much plastic becomes carelessly discarded, and end up in the world’s oceans.
Disposal, Fracturing, Accumulation and potential Marine Ecological Drivers
In 1997, what would become known as the “Great Pacific Garbage Patch”, was discovered in the North Pacific for the first time, spurring much scientific interest and concern in understanding this man-made phenomena (Larsen, 2014). With greater interest in the growth of production, and in use of plastic items, recent studies and literature reviews have attempted to determine the amounts of plastics which have come to reside in the ocean, what factors contribute to the generation and degradation of plastics in the ocean, and the effects that resulting microplastics have on marine ecosystem function.
Research by Barnes et al., (2009) has catalogued the build up of plastic debris in shoreline and marine environments worldwide, reporting that the overall amount of microplastic debris is increasing, as well as the area encompassed by such debris. Barnes and colleagues highlighted several non-point sources of plastic generation, pointing out that both macro and microplastic debris is dumped directly into the ocean by wastewater management facilities, fishing boats and cargo ships, through the release of plastic products from containers and gear being washed overboard, as well as from improperly buried plastics released from land fills. The researchers argue that much of the plastic debris which consists of items such as shopping bags, cellophane films, exfoliant micro-beads, and other light plastics, are easily degradable and are believed to most likely be sinking to the seabed. Their research reported on the dispersement of plastics in the oceans and shorelines, and reported unequal surface levels of plastic debris, with higher levels observed in the oceans of the northern hemisphere, compared to the oceans of the southern hemisphere. Albeit, quantifiable amounts were difficult to assess. However, it is known that industrialized nations such as the U.S.A. and China accounted for up to 16.3% and roughly 13% of national waste discarded as plastic, respectively in 2005 (US EPA 2006; World Bank 2005). Barnes and colleagues expressed concern towards the current prevalence of microplastics observed in the world’s oceans, as microplastic debris is expected only to increase in prevalence, the effects of which can last 1000 years or longer under certain conditions. Finally, the researchers pointed out and expressed concern that suspected fragmental behavior and the subsequent effects of microplastics on the marine environment were still not well-documented or understood.
A literature review conducted by Andrady (2011), discussed how plastics decompose, and how subsequent decomposition effects marine ecosystems. Andrady reported that macroplastic material decomposed through physical weathering via abrasive surfaces able to tear up the plastic, such as roadways and sand. The prevalence of this waste comes from land-based human consumption, accounting for 80% of microplastic waste (Watson et al., 2006), after which disintegration continues through the chemical processes of weathering and dissolving from the effects of photodegradation (via sunlight exposure), sorption by sea water, and the subsequent sorption by microbes, such as zooplanktons. Illustrating the effects of microplastic debris on marine biota, Andrady also discussed the behavior of free-floating chemical additives conglomerating around microplastics suspended in the ocean, which could subsequently be ingested from smaller to larger marine animals. Finally, due to the fractal nature and behavior of microplastics, Andrady emphasizes the need to quantify and determine the amount of microplastics in the ocean and bioavailability.
In a study conducted by Nikolai et al., (2012), the researchers created trajectory models to predict potential pathways and locations by which discarded plastics could reach five potential accumulation regions believed to exist as gyre convergence points in the world’s oceans. Their results predicted regions of accumulation of plastic debris in both the northern and southern Atlantic and Pacific oceans, as well as in the Indian Ocean. The researchers predicted differing concentrations projected to be found within and without the gyres of these oceans, with greater accumulation in the northern Atlantic and Pacific oceans (see fig. 1).
Figure 1 – Light and dark grey regions denote concentration zones of ocean surface plastic debris, with dark grey displaying central and light grey, non-central zones. The colored dots denote levels of accumulation.(Figure Source: Cozar et al., 2014; as cited from Nikolai et al., 2012)

In a subsequent study, original research was conducted by Cózar et al., (2014) in which researchers observed trends in sizes and accumulation of plastic debris during expeditions to the five known oceanic gyres. Based on sample sizes collected during their expeditions, the researchers hypothesized four ways by which an estimated 10,000 – 40,000 tons of ocean-surface plastic fell short of potentially far greater total amounts of plastic debris beneath and beyond the areas of the major gyres of the world’s oceans. Incorporating theories from research by Andrady, (2011), the researchers proposed that the “disappearance” of this plastic could be attributed to 1 or more of the following: Shore deposition, fragmentation, biofouling and/or ingestion. After the collection and sampling of plastic debris of varying sizes, the researcher’s hypothesis seemed supported that the true amount was again much greater, as the net introduction of plastic by industrialized nations did not account for the low surface amounts which their observations showed, and the high amounts that previous predictions suggested actually exists (Nikolai et al., 2012; Barnes et al., 2009). Analyses of the collections of plastic samples from the five ocean gyre surfaces showed most of the samples were equal to 2 mm or below 1 mm in size. Far smaller particles of plastic at the scale of only a few microns were believed to be to difficult to attain through the 200-μm mesh nets used, due to the fracturing behavior of microplastics towards ever-smaller size scales. These size samples suggested the ability of the sinking of such debris, and illustrated that a greater range of marine biota at all of the trophic levels could accidentally ingest such particles, causing the low surface levels observed, via bioaccumulation, and the suspected effects of defecation on the density of such sized debris. However, these methods of disappearance were not considered conclusive, as these mechanisms were again only shown to be possible by the researchers. Ultimately, the researchers confirmed the accumulation of plastic and microplastic debris in the five gyres as predicted to exist in all of the world’s oceans, and estimated that over 1/3 of all surface plastic debris has accumulated in the North Pacific Gyre, most likely due to the Pacific ocean being shared by the USA, China and Japan.
In relevant laboratory and observational studies, research results from two separate studies investigated possible mechanisms involved in the disappearance of microplastics from ocean surfaces. The respective studies provide some confirmation that the mechanisms of fragmentation and subsequent ingestion are likely to occur by marine organisms.
Research by Zettler and colleagues (2013), observed and recorded bacterial communities residing on plastic debris. The researchers collected samples from several regions of the North Atlantic. Observations made through electron microscopes suggested a symbiotic relationship between the bacteria and plastic debris, as the in-depth observations revealed indented boreholes in the shape of the bacteria. Subsequent rRNA genomic sequence analyses of the bacteria showed the ability for hydrocarbon polymer hydrolysis, giving strong support towards plastic debris becoming fragmented at truly microscopic scales, and providing evidence for the previous theories and observations of bioaccumulation being made possible through ingestion by larger marine biota.
In another laboratory study conducted by Ward & Kach, (2009), nanoparticles of chemical additives and microplastic beads of polystyrene plastic were shown to be ingested by marine bivalves, namely mussels and oysters in varying quantities. The amount ingested was related to the size of the particles and time of year, which when combined at proper sizes and time of year, resulted in high levels of ingestion at > 70% of the nanoparticles presented to the bivalves. The researchers pointed out that the chemicals and plastics that they presented to the bivalves in the experiment are found in sunscreens, paints, and wastewater discharge, among other domestic and industrial products. The researchers presented a likely pathway for the movement of toxicity up through the trophic levels and suggest significant amounts through ingestion by small marine organisms, make it into the human diet.
Finally, recent research conducted by Woodall et al., (2014) has been the first known study to confirm great quantities of microplastic items to amass in the deep sea, and provide estimates of the amount potentially dispersed across the world’s seabeds. The researchers collected deep-sea sediment cores from canyons, sea-mountains, basins and continental gradients up to depths of 3500 meters (11,400 feet) for samples of microplastic debris. The cores were collected from the North Atlantic and Indian Oceans, as well as from the Mediterranean Sea. Examining the contents of the cores collected, the researchers observed up to 40 microplastic items per 50 ml of sediment. Most of the debris they observed were in the form of small fragmented strands of 2-3 mm in length, and only .1 mm in diameter. The researchers conservatively estimated that within the Indian Ocean seabed alone, upwards of 4 billion microplastic fibers could exist per square kilometer. The researchers further estimated that the worldwide abundance of deep-sea microplastic debris exceeded the levels of ocean surface debris by four times the amount.
Toxicology and Biological Impacts of Microplastics
As recent research has shown, the microscopic size and prevalence of the greater abundance of plastics found in the world’s oceans allows for high chance of a vast array of marine biota to ingest the plastics for as long as microplastic debris is available in the oceans. It remains that research on the toxicity of microplastics be reviewed.
A critical literature analysis by Oehlmann and colleagues (2009) investigated prevalent chemicals used in the manufacturing of plastics and the toxicological effects observed in marine and terrestrial animals. Many chemical additives were referenced in the study, including Bisphenol A (BPA), butyl benzyl phthalate (BBP), and a plethora of related phthalate variants. BPA and various forms of phthalates are among some of the most prevalent additives used in manufactures. In the study it was discussed that the effects of these additives varied depending on the animal and type of synthetic polymer it was exposed to. For example, original research by the team showed that the sperm of the Zebrafish became sluggish in velocity when exposed to BBP and methanol. Other forms of phthalates discussed by the researchers had been shown to cause organ cell death, pre-natal developmental and chromosomal abnormalities in several species of mollusks and annelids (Dixon et al., 1999; Baršienė et al. 2006). Other studies have also shown the ability for acute phthalate absorption in fish (Staples et al., 1997), acting as endocrine disruptors, and subsequently effecting the production and hormonal uptake of androgens, causing physiological changes to sex organs (Liu et al, 2005).
Exposure to BPA has also been shown to cause the physiological morphing and cellular malformation of sex organs in mollusks (Aarab et al., 2006), such as mussels and oysters, as well as cell membrane damage (Canesi et al. 2005). BPA’s are known to contain synthetic estrogens, and research has reported that the added concentrations combined with natural estrogens have been found in fish exhibiting gynecomastic changes to reproductive organs even at low concentrations, and concentrations which have been found in field studies (Harris et al., 1997; Olea et al., 1996; Sonohi et al., 2001). Oehlmann and colleagues conclude by reiterating the harmful, quickly-induced, inhibiting effects on reproduction and development caused by phthalates and BPA on numerous species found in marine environments. The researchers expressed concern, and the need for further research, on the longterm effects of phthalates and BPA on marine biota.
Talsness and colleagues (2009) collected data on the effects of prevalent plastic additives such as Phthalates, BPA, polybrominated diphenol ethers (PBDE) and tetrabromobisphenol A (TBBPA), as these additives have also been detected in both lab animals and humans (Lang et al., 2008). Phthalates and BPA are among the most common compounds found in plastic products and are known to seep rapidly from products such as polyvinyl chloride (PVC), makeup products, plastic films, and “microwavable” containers, among others, especially when heated (Matsumoto et al., 2002; Bae et al., 2002). Research results on phthalate and BPA exposure at known human exposure levels were shown to cause genetic and phenotypical disorders in newborn and adult animals, with newborns being significantly more susceptible to neurological and physiological developmental maladies due to higher concentrations of such additives. General findings on adequate levels of phthalates in animals were correlated with testicular cancer, lack of sex organ development and reduction in functional efficiency. Findings on BPA exposure showed similar and other effects on many organs of the bodies of the animals involved. Such effects included induced onset of type 2 diabetes, disturbance of neural configurations of the hippocampus, and heart disease, among others (Alonso-Magdelena et al., 2005; MacLusky et al., 2005; Lang et al., 2008). In a study by Nagel et al. (1997), pregnant mice were fed a daily dose of BPA that was 25 times lower than the levels considered safe for human consumption by the FDA and other food safety authorities. The researchers observed many of the aforementioned adverse effects on the reproductive systems of the male offspring of the pregnant mice. As Talsness and colleagues reviewed literature on the compounds PBDE and TBBPA, some toxicological effects were similar with both compounds effecting thyroid function and development. TBBPA was found in heavy concentrations in human and animal breast milk (Thomsen et al., 2002), supporting high levels of estrogen in human breast cancer cells (Olsen et al., 2003). Exposure to PDBE has shown to negatively effect thyroid function, and thus neurodevelopment in animals and humans. Such vital developmental processes effected include neurogenesis, axonal thickness and sheathing, necessary protein synthesis for the cell repair of neurons, and impairment of learning and memory, among other neuronal sensitive skills (Haddow et al., 1999; Viberg et al., 2003, 2008). Through their research, Talsness et al., (2009) starkly illustrate and support a clear argument for necessary regulations to be made to decrease the exposure and ubiquity of these compounds, especially for humans in pre- and postnatal development.
An Issue of Law: They Don’t Make Regulations Like They Used To
In light of the recent research that has been reviewed thus far, it should be evident that international regulations such as that which resulted from the MARPOL 73/78 conventions do little to enforce global agreements to limit plastic debris input to the ocean. However, regulations are still most needed to outline such necessary procedures and mitigate the amounts of plastic and resulting microplastics consumed and discarded, for the sake of environmental and human health. Yet, results towards effective regulation do not always come to be. This section of the paper will discuss the difficulties of creating truly implementable regulation, as well as the policies and laws which have been enacted to mitigate plastic and microplastic levels.
Shaxson (2009) illustrates the relationships between researchers and policymakers in terms of creating effective policies involving the production, and discarding of plastics specifically in the UK. Research by Shaxson highlights the process of creating legislation and points to the dichotomy created during the legislation process by various groups (lobbyists) who vie for different (sometimes opposing) interests. Shaxson highlights four outcomes which can result from the interactions between scientist and policy makers, which include 1) Well-structured problems, 2) Moderately structured problems, 3) Badly structured problems, and 4) Unstructured problems. Of these four outcomes, the amount of relevant data is the key for understanding what legislation needs to be passed, for what purpose, and what improvement is expected.
In a well-structured scenario the environmental issue can be fully comprehended as to why the issue may have arisen in the first place, the evidence for a resolution to the issue is considered conclusive by both scientists and policy makers, and the benefits of change are believed to be in the interest of all sectors of economy, public health, etc. Then, legislation can easily be passed to ensure high quality living standards. In a moderately-structured scenario, the environmental issue and the necessity for policy change is clear, however, the methods for alleviating the issue remain inconclusive, with monetary costs and outdated or biased research may become the deciding factor for a best suited solution, as opposed to objective research. In a badly structured problem, even sound research may be ignored especially if it presents new data which has ‘strange’, previously unheard of implications which can be difficult for non-experts to understand. This can open the way for lobbyists to falsely vie for an interest which can be advertised in deceiving ways, and more comfortably accepted by politicians, such as an interest which can be touted as all natural, job-producing or “sustainable” (Turnhout et al., 2007). Finally, in an unstructured problem scenario, the environmental issue and why anything needs to be done about it is of no consideration as the evidence is not believed to be significant among politicians. Shaxson highlights the issue of chemical additives found in plastics as an unstructured issue due to current evidence on compounds such as phthalates and BPA being seen as having insignificant effects on human and animal health (vam Saal & Welshons, 2006; Oehlmann et al., 2009). Thus, while sure questions may be developed on what must be done to mitigate an issue such as microplastic ubiquity and subsequent effects, there may still exist questions and uncertainty from policy makers of the validity of a research group’s data, or the requirements for fixing the problems may be considered too challenging and stirring of a change to commit to. Thus, ambiguity continues over the best way to fix a problem, thus policy change can and does remain stagnant and environmental issues are further exacerbated.
Progressive Regulation: On a Positive Note
In an article by Larsen (2014), the author discusses the current laws surrounding the banning or taxing of the plastic grocery bag. Within the last 25 years legislation has been passed to ban or tax plastic bags by cities, states and countries around the world. Such cities as Washington D.C. have placed a 5 cent tax on the use of plastic shopping bags, whereas other cities such as Austin, Texas and many retailers within Seattle, WA have banned the use altogether. Honolulu County, HI, and the state of California have also banned the use of plastic bags. However, some of these legislations will not go into effect until 2015, and efforts by the nations of Denmark, China, and Ireland have seen relative success in plastic bag consumption through taxing, while Bangladesh remains the only nation to ban plastic bags altogether. In total, roughly 15% of American citizens will soon no longer have the option or have to pay to use disposable plastic shopping bags (see fig. 2).
Figure 2 – Number of people (in millions) who have been and will be effected by plastic bag legislation. (Figure Source: Earth Policy Institute, 2014.
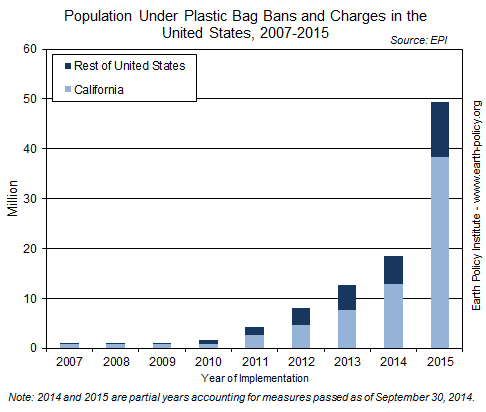
Alternatives to Plastics and Potential for Mitigation of Macro and Microplastic Debris
In a review by Song et al., (2009) renewable alternatives for plastics are discussed in depth, citing recent research showing the possibility for such alternatives to be mass produced at similar scales to conventional plastics used today. According to the researchers, specific qualifications must be met to be a considerable biodegradable plastic, including the materials used, the breakdown rate, as well as the elements that the biodegradable plastics breaks down into. Biodegradable plastics have been and are being made from cornstarch, sugar, silk, collagens, and other plant and animal based renewable material, which can can deteriorate into carbon dioxide, water and disintegrating biomass which can be utilized by bacterium to form fully non-toxic biomass (soil). Despite the potential for the environmental compatibility and similarities in the range of use that biodegradable plastics hold, higher economic costs at two to 4 times the price of conventional plastic manufacturing keep production low at roughly 350,000 tons per year (Bioplastics 07/08). Furthermore, since biodegradable plastics are accepted by conventional waste municipalities, they are treated similarly, ending up as fuel for incineration, and landfill waste, in which concern over high levels of methane may be produced, contributing to GHG emissions (Hudgins, 1999). However, household and municipal composting systems are used, but with varying degrees of success, as temperature and allotted time lapse requirements for compostability are not always met, and differing types of biodegradable plastics require different conditions, which is not widely known or understood by the general public (Thompson et al., 2009b). Regardless, the potential and current use in consumer products are promising, and further development of truly sustainable and biodegradable plastics may be the future in an effort to mitigate the origination of plastic and subsequent microplastics into the environment.
As may be obvious, the scale of the issue of microplastics is not fully understood until efforts have been made showing significant results in mitigation of the plastic debris in the ocean. Innovative, independently funded, large scale clean up projects, such as The Ocean Clean Up Project, founded by Boyan Slat, have been underway in hopes of clearing the ocean surface of plastic debris. In a detailed report by Slat and colleagues (2014) discussing the purposes, aims, logistics, methods and probable outcomes of the Ocean Clean Up Project, the authors point out that conventional methods for cleaning the world’s gyres would demand billions of dollars over thousands of years to clean the surfaces of the ocean’s gyres by collecting plastic debris with nets dragged by ships (Moore, 2011). The rate of collecting and discarding of plastic waste would virtually cancel one another out. Rather, the innovative idea to allow the flow of the oceanic gyres (namely, the North Pacific Gyre) to transport the plastic debris against strategically placed anchored and boom-suspended barricades beneath the ocean surface was favored. Preliminary modeling and field research by the group has determined that a barricade draft of 3 meters, at 100 km in length was sufficient to capture 42% of the plastic floating in the North Pacific Gyre in 10 years. The total costs have been estimated at roughly 317 million euro over the estimated 10 years of operation. Post-collection transportation would be handled by barges, or catamaran-hulled ships for the separation processing of the plastic debris. The Ocean Clean Up Project has demonstrated the ability of reusing some of the debris as pyrolysis oil, created from the separated and highly heated polyolefin plastics. The characteristics of the pyrolysis oil was found to be similar to unprocessed crude oil. The well-funded, institutionally supported Ocean Clean Up Project shows promising success as an environmental enterprise expected to be near full operation by 2017. The project is no small feat, however, the aim of the project concentrates on ocean surface plastic debris, with only surface plastic being collected, recycled and reused. Thus, deep sea plastic remains an issue, as it continues to persist in the environment.
General Conclusions and Implications
It is the hope of the author that the research that has been reviewed in this paper on the issues of microplastics prevalence and the toxicological effects has illustrated the urgency of the matter, and highlighted the need for cooperation and development in the political, economical and technological sectors to ensure the future health of the oceans and people. Most issues surrounding microplastics are well understood, but resolving the issue of microplastics does not seem like it may be a straight-forward process, as the issue of deep sea plastic will most likely remain for as long as plastic is dumped and degraded in the oceans, and for as long as concerted efforts and technology take to mitigate it. Current policies on plastic production, consumption and disposal suggest policies at an international level do not seem to reflect the urgency of the matter that the decomposition of plastic items into microplastics pose to human and environmental health. However, new yet broadly worded policies and regulations on plastics production and disposal at national and international levels will not necessarily guarantee a reduction in the quantities of plastics entering the oceans as enforcement becomes to difficult at this scale. Due to an inability to point to sources of plastic pollution, municipalities and companies will likely remain unhindered in the methods by which business is conducted. However, state and city bans on plastic bags seem to hold more promise for the mitigation of plastic items entering the environment, as regulations at this scale can be easier to oversee by community and state regulation committees. Ultimately, it should be clear, as the literature points out, that plastic is everywhere in our lives, and plastic bags are not the only source for the origination of microplastics and are only a small fraction of the problem. Our modern age has become increasingly dependent on the conveniences of plastic items and plastic technology in all sectors of daily life, with our dependency expected to grow in the coming decades. Thus, the analysis of current research provided in this paper may imply that operations for the proper disposal and reclamation of plastic items and microplastic byproducts from marine habitats maybe be best suited for success with smaller scaled, independently funded, state or county projects. This would likely allow for stronger oversight and implementation of technologies and effective clean up efforts with local parties assessing and executing microplastic elimination projects.
Featured Image Copyright: 123RF Stock Photo
References
Aarab N., Lemaire-Gony S., Unruh E., Hansen P. D., Larsen B. K., Andersen O. K., Narbonne J. F. 2006. Preliminary study of responses in mussel (Mytilus edulis) exposed to bisphenol A, diallyl phthalate and tetrabromodiphenyl ether. Aquat. Toxicol. 78, 86–92 (doi:10.1016/j.aquatox.2006.02.021).
Alonso-Magdalena, P., Morimoto, S., Ripoll, C., Fuentes, E. & Nadal, A. 2006 The estrogenic effect of bisphenol A disrupts pancreatic beta-cell function in vivo and induces insulin resistance. Environ. Health Perspect. 114, 106 – 112.
Andrady, A. L. (2011). Microplastics in the Marine Environment. Marine Pollution Bulletin, 62(8), 1596–1603. doi:10.1016/j.marpolbul.2011.05.030
Bae, B., Jeong, J. H. & Lee, S. J. 2002 The quantification and characterization of endocrine disruptor bisphenol-A leaching from epoxy resin. Water Sci. Technol. 46, 381–387.
Barnes, D. K., Galgani, F., Thompson, R. C., & Barlaz, M. (2009). Accumulation and fragmentation of plastic debris in global environments. Phil. Trans. R. Soc. B, 364(1526), 1985–1998. doi:10.1098/rstb.2008.0205
Baršienė J., Šyvokienė J., Bjornstad A. 2006. Induction of micronuclei and other nuclear abnormalities in mussels exposed to bisphenol A, diallyl phthalate and tetrabromodiphenyl ether-47. Aquat. Toxicol. 78, 105–108 (doi:10.1016/j.aquatox.2006.02.023)
Bioplastics 07/08 Processing parameters and technical characteristics—a global overview, Bioplastics24.com., ISSN 1863-7299. Slat, B. (2014). How The Oceans Can Clean Themselves: A Feasibility Study. The Netherlands: The Ocean Cleanup. Retrieved from http://www.theoceancleanup.com/fileadmin/media-archive/theoceancleanup/press/downloads/TOC_Feasibility_study_lowres_V2_0.pdf
Canesi L., Betti M., Lorussoa L. C., Ciaccia C., Gallob G. 2005. ‘In vivo’ effects of bisphenol A in Mytilus hemocytes: modulation of kinase-mediated signalling pathways. Aquat. Toxicol. 71, 73–84 (doi:10.1016/j.aquatox.2004.10.011)
Cózar, A., Echevarría, F., Duarte, C. M., Irigoien, X., Úbeda, B., Hernández-León, S., Palma, Á. T., et al. (2014). Plastic debris in the open ocean. PNAS, 111(28), 10239–10244. doi:10.1073/pnas.1314705111
Dixon D. R., Wilson J. T., Pascoe P. L., Parry J. M. 1999. Anaphase aberrations in the embryos of the marine tubeworm Pomatoceros lamarckii (Polychaeta: Serpulidae): a new in vivo test assay for detecting aneugens and clastogens in the marine environment. Mutagenesis 14, 375–383 (doi:10.1093/mutage/14.4.375)
Haddow, J. E. et al. 1999 Maternal thyroid deficiency during pregnancy and subsequent neuropsychological develop- ment of the child. N. Engl. J. Med. 341, 549–555. (doi:10.1056/NEJM199908193410801)
Harris C. A., Henttu P., Parker M. G., Sumpter J. P. 1997. The estrogenic activity of phthalate esters in vitro. Environ. Health Perspect. 105, 802–811 (doi:10.2307/3433697)
Hudgins, M. 1999 Aerobic landfill studies from the USA. Paper presented at the 1st Int. Conf. Solid Waste, April 1999, Rome.
Lang, I. A., Galloway, T. S., Scarlett, A., Henley, W. E., Depledge, M., Wallace, R. B. & Melzer, D. 2008 Association of urinary bisphenol A concentration with medical disorders and laboratory abnormalities in adults. JAMA 300, 1303 – 1310. (doi:10.1001/jama.300.11.1303)
Larsen, J. (2014). Plastic Bag Bans or Fees Cover 49 Million Americans. Retrieved from http://www.earth-policy.org/data_highlights/2014/highlights49
Liu K. J., Lehmann K. P., Sar M., Young S. S., Gaido K. W. 2005. Gene expression profiling following in utero exposure to phthalate esters reveals new gene targets in the etiology of testicular dysgenesis. Biol. Reprod. 73, 180–192 (doi:10.1095/biolreprod.104.039404)
MacLusky, N. J., Hajszan, T. & Leranth, C. 2005 The environmental estrogen bisphenol A inhibits estrogen- induced hippocampal synaptogenesis. Environ. Health Perspect. 113, 675–679.
MARPOL, International Convention for the Prevention of Pollution from Ships (1973). International Maritime Organization Retrieved from http://www.imo.org/About/ Conventions/ListOfConventions/Pages/International-Convention-for-the-Prevention-of-Pollution-from-Ships-(MARPOL).aspx.
Matsumoto, J., Yokota, H. & Yuasa, A. 2002 Developmental increases in rat hepatic microsomal UDP-glucuronosyl- transferase activities toward xenoestrogens and decreases during pregnancy. Environ. Health Perspect. 110, 193 – 196.
Moore, C. J., & Phillips, C. (2011). Plastic Ocean: How a Sea Captain’s Chance Discovery Launched a Determined Quest to Save the Oceans: Avery Books.
Nagel, S. C., vom Saal, F. S., Thayer, K. A., Dhar, M. G., Boechler, M. & Welshons, W. V. 1997. Relative binding affinity-serum modified access (RBA-SMA) assay pre- dicts the relative in vivo bioactivity of the xenoestrogens bisphenol A and octylphenol. Environ. Health Perspect. 105, 70–76. (doi:10.2307/3433065)
Nikolai, M., Jan, H., & Peter, N. (2012). Pathways of marine debris derived from trajectories of Lagrangian drifters. Marine Pollution Bulletin, 65(1-3), 51–62. doi:10.1016/j.marpolbul. 2011.04.016
Oehlmann, J. et al. 2009 A critical analysis of the biological impacts of plasticizers on wildlife. Phil. Trans. R. Soc. B 364, 2047–2062. (doi:10.1098/rstb.2008.0242)
Olea N., Pulgar R., Perez P., Oleaserrano F., Rivas A., NovilloFertrell A., Pedraza V., Soto A. M., Sonnenschein C. 1996. Estrogenicity of resin-based composites and sealants used in dentistry. Environ. Health Perspect. 104, 298–305 (doi:10.2307/3432888)
Olsen, C. M., Meussen-Elholm, E. T. M., Samuelsen, M., Holme, J. A. & Hongslo, J. K. 2003. Effects of the environmental oestrogens bisphenol A, tetrachlorobisphenol A, tetrabromobisphenol A, 4-hydroxybiphenyl and 4,40 -dihydroxybiphenyl on oestrogen receptor binding, cell proliferation and regulation of oestrogen sensitive proteins in the human breast cancer cell line MCF-7. Pharmacol. Toxicol. 92, 180–188. (doi:10.1034/j.1600-0773.2003.920408.x)
Plastics-the facts 2014. (2014). Retrieved from http://issuu.com/plasticseuropeebook/docs/final_plastics_the_facts_2014_19122?e=14847134/1062116
Shaxson, L. (2009) Structuring policy problems for plastics, the environment and human health: reflections from the UK. Phil. Trans. R. Soc. B 364, 2141–2151. doi:10. 1098/rstb. 2008.0283
Song, J. H., Murphy, R. J., Narayan, R. & Davies, G. B. H. 2009 Biodegradable and compostable alternatives to conventional plastics. Phil. Trans. R. Soc. B 364, 2127–2139. (doi: 10.1098/rstb.2008.0289
Sohoni P., et al. 2001. Reproductive effects of long-term exposure to bisphenol A in the fathead minnow (Pimephales promelas). Environ. Sci. Technol. 35, 2917–2925 (doi:10.1021/es000198n)
Staples C. A., Peterson D. R., Parkerton T. F., Adams W. J. 1997. The environmental fate of phthalates esters: a literature review. Chemosphere 35, 667–749.
Talsness, C. E., Andrade, A. J. M., Kuriyama, S. N., Taylor, J. A. & vom Saal, F. S. 2009 Components of plastic: experimental studies in animals and relevance for human health. Phil. Trans. R. Soc. B 364, 2079– 2096. (doi:10.1098/rstb.2008.0281)
Thompson, R. C., Swan, S. H., Moore, C. J., & vom Saal, F. S. (2009a). Our Plastic Age. Phil. Trans. R. Soc. B, 364, 1973–1976. doi:10.1098/rstb.2009.0054
Thompson, R. C., Moore, C. J., vom Saal, F. S. & Swan, S. H. (2009b). Plastics, the environment and human health: current consensus and future trends. Phil. Trans. R. Soc. B 364, 2153–2166. (doi:10.1098/rstb.2009.0053)
Thomsen, C., Leknes, H., Lundanes, E. & Becher, G. 2002 A new method for determination of halogenated flame retardants in human milk using solid-phase extraction. J. Anal. Toxicol. 26, 129–137.
Turnhout, E., Hisschemoller, M. & Eijsackers, H. 2007 Ecological indicators: between the two fires of science and policy. Ecol. Indic. 7, 215–228.
US EPA 2006 Municipal Solid Waste in the United States: 2005 facts and figures. EPA530-R-06-011, United States Environmental Protection Agency, Office of Solid Waste, Washington, DC.
Viberg, H., Fredriksson, A. & Eriksson, P. 2003 Neonatal exposure to polybrominated diphenyl ether (PBDE 153) disrupts spontaneous behaviour, impairs learning and memory, and decreases hippocampal cholinergic receptors in adult mice. Toxicol. Appl. Pharmacol. 192, 95–106 (doi:10.1016/S0041-008X(03)00217-5)
Viberg, H., Mundy, W. & Eriksson, P. 2008 Neonatal exposure to decabrominated diphenyl ether (PBDE 209) results in changes in BDNF, CaMKII and GAP- 43, biochemical substrates of neuronal survival, growth, and synaptogenesis. Neurotoxicology 29, 152 – 159. (doi:10.1016/j.neuro.2007.10.007)
vom Saal, F. & Welshons, W. 2006 Large effects from small exposures. II. The importance of positive controls in low-dose research on bisphenol A. Environ. Res. 100, 50–76.
Ward, J.E., Kach, D.J., 2009. Marine aggregates facilitate ingestion of nanoparticles by suspension-feeding bivalves. Mar. Environ. Res. 68 (3), 137–142.
Watson, R., Revenga, C., Kura, Y., 2006. Fishing gear associated with global marine catches I. Database development. Fish. Res. 79 (1–2), 97–102
Woodall, L. C., Sanchez-Vidal, A., Canals, M., Paterson, G. L., Coppock, R., Sleight, V., Calafat, A., et al. (2014). The deep sea is a major sink for microplastic debris. R. Soc. open sci., 1(4), 1–9. Retrieved from http://dx.doi.org/10.1098/rsos.140317
World Bank 2005 Waste Management in China: issues and recommendations. Working Paper No. 9, Urban Development Working Papers, East Asia Infrastructure Development.
Zettler, E. R., Mincer, T. J., & Amaral-Zettler, L. A. (2013). Life in the “Plastisphere”: Microbial Communities on Plastic Marine Debris. Environmental Science & Technology, 47(13), 7137–7146. doi:10.1021/es401288x